Natural Sciences
Evolving Idea
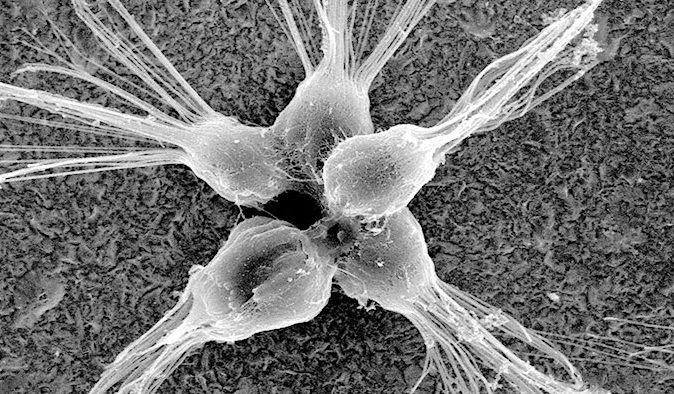
Ken Prehoda has been getting a lot of email and letters lately. This sort of thing happens when you make breakthroughs in our understanding of evolution.
“This has been fun for me,” the UO biochemist said in an interview, as he recapped a recent talk on his latest research. “I’ve gotten lots of feedback from the public. People have a lot of really good questions.”
It’s been a wild ride for Prehoda. He bookended 2016 with discoveries that illuminate the rise of multicellular life in humans and other animals.
First, the biochemist published a finding that swept through the field of cell biology and landed on the pages of The New York Times and The Washington Post: Prehoda had identified a genetic change important for launching multicellular life.
The theory of evolution tells us that, despite all our multicellular complexity, we come from a single-celled ancestor. So how did we get from there to here?
Working with colleagues at the University of California at Berkeley, Prehoda examined our closest living relatives among single-celled organisms. Choanoflagellates (right) are sponge-like creatures that live in the sea; they intrigued Prehoda because they also exist in colonies, a halfway point of sorts between single-cell and multicellular life.
Next came a bit of time travel. With the sequence of genes in choanoflagellates and other organisms at his disposal, Prehoda’s lab collaborated with colleagues at the University of Chicago to make extrapolations about the molecular changes that had led to these genes hundreds of millions of years ago.
His discovery centered on proteins, the workhorses in cells that perform jobs such as speeding up chemical reactions. Prehoda found a particular protein that switched from accelerating chemical reactions to binding with other proteins—just the thing for sticking one cell to another, and then another, and so on.
Even more surprising: This wasn’t an evolution that played out slowly over time. It happened in a single, random mutation.
“That was the really shocking finding,” Prehoda said. “This is really a dramatic functional jump.”
He followed it up late in the year, this time working with colleagues at the Medical College of Wisconsin. Researchers learned the process by which that special protein had evolved so that it could bind with others—basically, how it became a scaffold upon which other “molecular machinery” could be built. With the mutation, the protein moved from being pliable to much more rigid, and that was crucial for this new function.
“Our protein, before the mutation, was an enzyme that had certain flexible movements,” Prehoda said. “This one mutation fixed the protein’s backbone, locking the molecule into a shape that is important for its new function.”
The discoveries advance our understanding of evolution. They also provide a new way to look at diseases such as cancer, a condition in which damaged cells in our bodies no longer cooperate with others and instead act on their own as single cells—precisely the process that Prehoda has demystified, in reverse.
“Thinking about disease as a reversal of the evolutionary process is a new approach,” Prehoda said. “That change of perspective can have a huge impact in research, not to mention possible treatments.”
—Matt Cooper
Photo credit: Mark Dayel (Mark@Dayel.com)