Natural Sciences
Good Vibrations
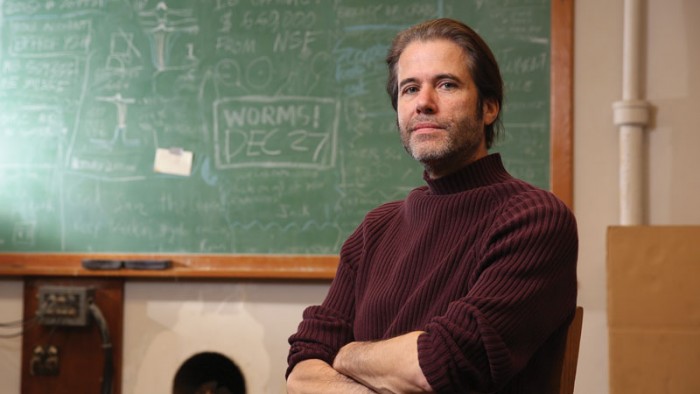
It’s been called one of the biggest breakthroughs in physics in 100 years. And it happened because UO scientist Robert Schofield (above) decided, at 4 a.m. last Sept. 14, to get some sleep.
Schofield was in a space observatory in Louisiana, working on what had been an abiding passion for physicists for decades: trying to prove the existence of “gravitational waves.” The theory, predicted by Albert Einstein a century ago, was that these energy ripples move across the cosmos, causing space and time to expand and contract.
No one had ever detected a gravitational wave. But on that fateful morning, Schofield and scientist Anamaria Effler opted to end a long night’s work; instead of making another test of the instrument designed to detect a gravitational wave, they set it up for observation and left.
Fifty-one minutes later, it happened: The system detected a gravitational wave that had been produced by the collision of two black holes a billion light-years away.
Physicists announced the discovery earlier this year, sending waves of another sort—shock and excitement—throughout the scientific community and beyond. The proof of gravitational waves validated a fundamental tenet of Einstein’s theory of relativity and triggered speculation that a Nobel Prize is on the way.
(What happens when black holes collide? Listen to physicist Ray Frey describe the stellar phenomenon and what it means for science.)
A BILLION-TRILLION SUNS
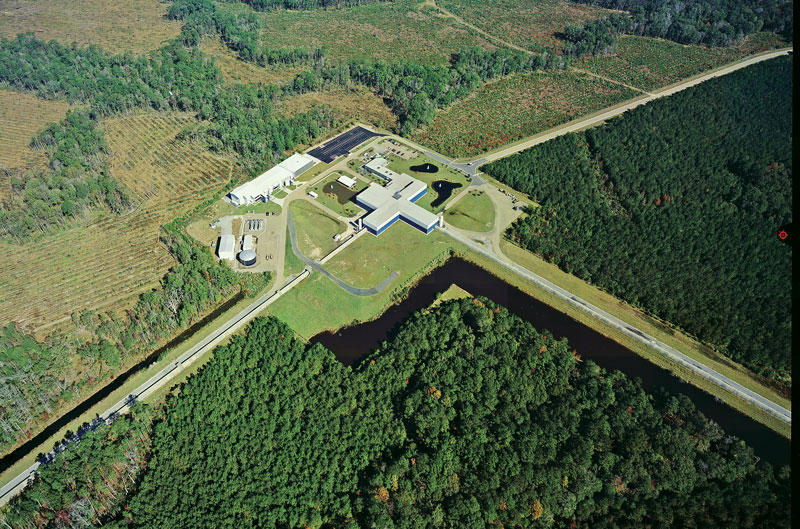
The Laser Interferometer Gravitational Wave Observatory
Details surrounding the discovery are simply staggering.
Consider, for example, the instruments that “heard” this gravitational wave: The Laser Interferometer Gravitational Wave Observatory (right) where Schofield was working, and another like it in Washington state, has L-shaped antennas with arms that each stretch 2.5 miles across the land. Their sensitivity to vibration is such that they have to filter out readings from water going over a dam 18 miles away.
Before impact, the black holes were circling each other at 250 times per second. The power surge that erupted from their collision had the energy of a billion-trillion suns—that power was 50 times greater than the output of all the stars in the universe. This surge moved across space at the speed of light.
And yet, the gravitational wave was so weak by the time it reached the observatories that it was captured as a vibration measuring just a fraction of the width of a proton.
That’s where Schofield and a team of UO physicists come in. Their job was to make sure that the passing “bump” had indeed been a gravitational wave, and not something else.
The observatory’s antennas are basically a system of mirrors that are suspended—perfectly still—within a chamber. By running constant beams of laser light between the mirrors, scientists can see whether a passing vibration jostles the mirrors even slightly—that throws the beams out of alignment, and that’s something that scientists can measure to determine the size and nature of the disruption.
The chambers enclose the mirrors in a vacuum, free from disturbances in the air that can affect measurements. But they still register vibrations triggered by earthquakes, trucks on a highway, lightning strikes and more.
In this project funded by the National Science Foundation and involving 1,000 scientists around the world, the UO is a founding member that monitors the environment for events that could trick the observatory’s instruments. This is done using a variety of specialized sensors that have been installed at the two sites under Schofield’s direction.
WHEN LIGHTNING STRIKES
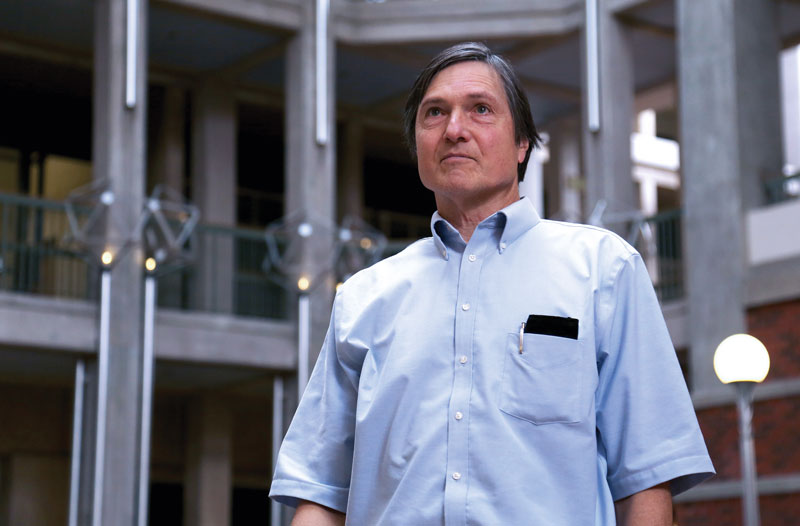
Ray Frey, physics department head, said that when the discovery was made, “we had our jaws on the ground for a while.”
Schofield—an expert at filtering out interference—is the project’s “go-to guy” for ruling out false positives, said Ray Frey (left), physics department head and leader of the UO team.
After the vibration was detected, scientists set about eliminating other possible causes.
In fact, at about the same time as the gravitational wave’s arrival, a massive lightning burst occurred over Burkina Faso in Africa. Some worried that the resulting electromagnetic wave, rather than a gravitational wave from deep space, had been recorded.
But Schofield has spent more than 15 years learning how lightning strikes affect the observatories’ antennas.
He determined that the strike—and 59 others around the world that happened in the second of the gravitational wave’s passing—was too small to be the culprit. Schofield went even further, tracking 11 strikes later in the year that were bigger and much closer to the antennas, and none of them registered as gravitational waves.
In a final report that allowed scientists to move forward with this momentous announcement, Schofield found no signal interference from anywhere on Earth.
The breakthrough creates new inroads in astrophysics and astronomy—scientists now have proven technology not just for viewing outer space, but listening to it.
The discovery illustrates “why we need to keep doing basic research,” Frey said. “It opens up a new way of looking at the universe. We’re really just looking forward to walking through that door and seeing what’s on the other side. This is just the start.”
Schofield called the find a victory for experimental physics and the kind of resource-heavy breakthrough that will be increasingly difficult to repeat without adequate federal funding.
He was awed that a collision in a very distant galaxy could cause detectable motions on Earth. But as for whether he regretted not being on hand at that instant when the universe reached out and touched us, Schofield was pragmatic.
“If I had been in the control room, I wouldn’t have seen it. It lasted one-third of a second,” he said. “I was glad to be at my motel. It was pretty late.”
—Matt Cooper